Dynamics and distribution of CO2 in karst vadose and epiphreatic zone (CARDIKARST)
Principal Investigator at ZRC SAZU
Franci Gabrovšek, PhD-
Original Title
Dynamics and distribution of CO2 in karst vadose and epiphreatic zone (CARDIKARST)
Project Team
dr. Cyril Mayaud-
Project ID
J7-4630
-
Duration
1 October 2022–30 September 2025 -
Lead Partner
Karst Research Institute Scientific Research Centre of Slovenian Academy of Sci…
-
Project Leader
-
Financial Source
Javna agencija za znanstvenoraziskovalno in inovacijsko dejavnost Republike Slovenije (ARIS)
Hrvatska zaklada za znanost
Karst areas are characterised by distinct surface and subsurface features created by the dissolution of carbonate rock in natural water. About 15% of the world's ice‐free continental area and nearly 22% of Europe are covered by karstifiable carbonate rocks (Goldscheider et al., 2020). Such proportions of potential carbonate weathering have attracted much attention in the study of the global carbon cycle and potential missing sinks (Liu et al., 2018). Karstification of carbonate areas is largely determined by an abundance of water and CO2, which control the dissolution and precipitation of carbonates. The complexity of karst structures is also reflected in the complexity of CO2 dynamics. The major sources of CO2 in karst are in the upper karst zones, the soil, and the epikarst, where CO2 is produced by biogenic respiration of roots or microbial activity and by decay of organic matter. While some CO2 is returned to the atmosphere, a proportion is transported further down into the vadose zone, either in dissolved form by infiltrating water or in gaseous form by diffusion and advection. During transport through karst zones, CO2 undergoes several transformations. When dissolved in water, it contributes to the dissolution of carbonates, consuming one mole of CO2 for each mole of dissolved calcite, thus, acting as a net CO2 sink. However, when water encounters well‐ventilated caves with lower CO2 levels than in the surrounding vadose zone, precipitation of calcite is triggered, with one mole of CO2 returning to the cave air for every mole of calcite precipitated. Some of this CO2 is then returned to the outside atmosphere through natural ventilation. In principle, these processes are well understood and researched, particularly in relation to the growth of speleothems, which have become one of the most attractive archives for reconstruction of past climate (Fairchild and Baker, 2012).
However, the effects of natural ventilation on the scale of a karst massif (and a CO2 reservoir in the vadose karst zone) are still beyond the reach of current research, despite its importance. Moreover, the interaction of the vadose CO2 reservoir with groundwater in the epiphreatic zone is still relatively poorly understood. CO2 exchange between groundwater and the vadose zone works in both directions. The vadose CO2 can dissolve into the groundwater and promote further calcite dissolution and the development of conduits. However, if the epiphreatic zone is well ventilated and has a low CO2 content, the exchange reverses; the CO2 degasses, which can lead to precipitation of calcite (White, 2013). An additional complexity involves interactions with the biosphere, for example where dissolved carbon can be captured by aquatic plants, which may result in relative long‐term storage of carbon. Although these processes are conceptually understood, only a limited number of observations have been made with simultaneous measurements in water and air (Covington et al., 2021).
The proposed project will investigate the following objectives:
1) Spatial and temporal heterogeneity of CO2 concentration in the vadose zone – its relationship with the structure of the vadose zone, external weather changes and natural ventilation.
2) CO2 exchange between air and groundwater in the epiphreatic zone – its magnitude and importance for speleogenesis and carbon fluxes at the catchment scale.
3) Size and nature of the karst carbon reservoir – observation‐based estimation of CO2 storage in the vadose zone of karst and assessment of carbon fluxes through a given karst system.
4) Development of methodology, measurement and observation techniques, establishment of a publicly available environmental database, and public outreach.
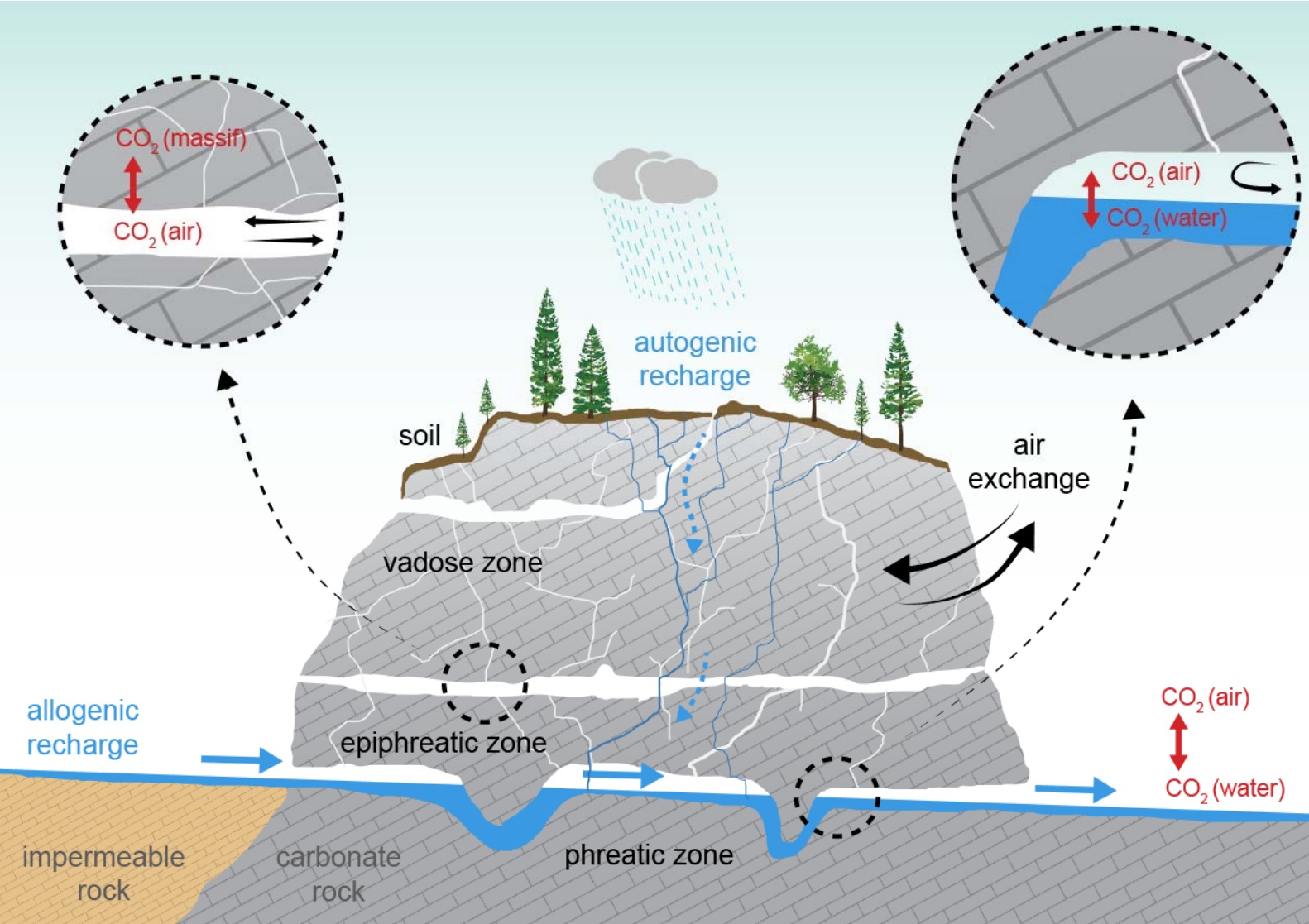
The work programme will consist of four intertwined work packages: Organisation and management (WP0), field activities and data acquisition (WP1), data analysis and modelling (WP2) and dissemination (WP3).
WP0 consists of management and dissemination actions. Dissemination will not target only scientists, but also students at all levels, park managers and general public. Both teams have experience and skills to promote science. The members of the Slovenian and Croatian groups have worked together in previous bilateral (exchange) programmes. They have co‐mentored students and have established a friendly and professional relationship from which this project will certainly benefit. We are all experienced cavers and have established good relationships with park and cave managers.
WP1 includes all field activities, installation and maintenance of monitoring stations. These activities are critical for the success of the project. We all have more than 15 years of experience in setting up observations and measurements in harsh cave environments.
Measurements of microclimate (including CO2) in the vadose zone are already underway in some of the caves. This basic set of automated stations will be maintained during the project and expanded to include new sites. To capture the heterogeneity of CO2 in the vadose zone, a suite of low‐cost instruments will be produced. Here, we will benefit from the experience of the Greek and U.S. collaborative groups that have already fabricated and deployed similar instruments. The epiphreatic zone will be studied by making (1) hydrological measurements (discharge) and (2) chemical measurements (water temperature, SEC and concentrations of major ions) and CO2 measurements in water and air. Water temperature and SEC will be recorded continuously, while major ion concentration will be determined occasionally by collecting water samples and conducting laboratory analyses. Monitoring in the epiphreatic zone is challenging. The zone is frequently flooded, so all instruments must be watertight and/or placed in a location that cannot be reached by high water. Instruments used for hydrologic studies, such as pressure, temperature, and conductivity loggers, can be several dozen metres underwater. Dissolved CO2 is measured with a new generation of CO2 sensors that can be submerged in water. Both continuous and point measurements will be made.
WP2 includes numerical modelling. Modelling activities are closely linked to data analysis and interpretation. Two types of model will be used. 1) A model of ventilation and gas exchange in the vadose zone, which is used to gain a basic understanding of how external climate, vadose zone structure, and conduit‐matrix exchange determine CO2 fluxes and heterogeneity. The model of ventilation has been developed within past projects and is ready for upgrade and implementation. 2) A model of cave evolution under turbulent water conditions will be used to evaluate the importance of air–water CO2 exchange on CO2 fluxes within the massif and the speleogenetic role of the exchange. The second model was developed by a research group at the University of Arkansas (USA) and is available to us for use in this project.
WP3 will deal with the results obtained in both WP1 and WP2. By combining data analysis and modelling results, we will produce a quantified model of CO2/carbon flux within the selected massif based on a solid understanding of the underlying processes. This study has the advantage of covering a large geographic area comprising different climatic, hydrological, and environmental conditions. Both the karst areas of Slovenia and Croatia are part of the Dinarides, hence, permitting the selection of diverse environments while retaining other parameters relatively constant. Subsequently, to better understand the vadose CO2 reservoir of karst massifs as a whole, this study will go beyond local constraints and try to merge existing worldwide cave monitoring data from caves with different geological, climatic and hydrological conditions. In this way, we will decipher which factors (e.g., hydrologic input, soil properties, temperature, and vegetation) have the most significant control on the extent and dynamics of the CO2 reservoir in the karst system. This can be accomplished by (1) making comparisons from a large number of caves, (2) performing a meta‐analysis of existing published data, and (3) synthesising data in the form of a geodatabase that can be used in the GIS software. A similar approach on a global scale has already been successfully implemented in speleothem science (e.g., SISAL ‐ Speleothem Isotopes Synthesis and AnaLysis and is being expanded to include cave monitoring metadata (cave‐ monitoring.org).
Atsawawaranunt, K., Comas‐bru, L., Mozhdehi, S.A., Deininger, M., 2018. The SISAL database: a global resource to document oxygen and carbon isotope records from speleothems 1687–1713.
Baldini, J.U.L., Bertram, R.A., Ridley, H.E., 2018. A first approximation of the Earth’s second largest reservoir of carbon dioxide gas. Sci. Total Environ. 616–617, 1007–1013.
Benavente, J., Vadillo, I., Carrasco, F., Soler, A., Liñán, C., Moral, F., 2010. Air carbon dioxide contents in the vadose zone of a Mediterranean karst. Vadose Zo. J. 9, 126.
Benavente, J., Vadillo, I., Liñán, C., del Rosal, Y., Carrasco, F., 2015. Influence of the ventilation of a karst show cave on the surrounding vadose CO2 reservoir (Nerja, South Spain). Environ. Earth Sci. 74, 7731–7740.
Bergel, S.J., Carlson, P.E., Larson, T.E., Wood, C.T., Johnson, K.R., Banner, J.L., Breecker, D.O., 2017. Constraining the subsoil carbon source to cave‐air CO2 and speleothem calcite in central Texas. Geochim. Cosmochim. Acta 217, 112–127.
Blackstock, J.M., Covington, M.D., Perne, M., Myre, J.M., 2019. Monitoring Atmospheric, Soil, and Dissolved CO2 Using a Low‐Cost, Arduino Monitoring Platform (CO2‐LAMP): Theory, Fabrication, and Operation. Front. Earth Sci. 7, 1–19.
Blatnik, M., Mayaud, C., Gabrovšek, F., 2019. Groundwater dynamics between planinsko polje and springs of the ljubljanica river, Slovenia. Acta Carsologica 48, 199–226.
Bourges, F., Genty, D., Perrier, F., Lartiges, B., Régnier, É., François, A., Leplat, J., Touron, S., Bousta, F., Massault, M., Delmotte, M., Dumoulin, J.P., Girault, F., Ramonet, M., Chauveau, C., Rodrigues, P., 2020. Hydrogeological control on carbon dioxide input into the atmosphere of the Chauvet‐Pont d’Arc cave. Sci. Total Environ. 716.
Covington, M.D., Gabrovšek, F., 2020. Modeling the impacts of cave ventilation and CO2 dynamics on speleogenesis, in: EGU General Assembly Conference Abstracts, EGU General Assembly Conference Abstracts. p. 18738.
Covington, M.D., Knierim, K.J., Young, H.A., Rodriguez, J., Gnoza, H.G., 2021. The impact of ventilation patterns on calcite dissolution rates within karst conduits. J. Hydrol. 593, 125824.
Covington, M.D., Prelovšek, M., Gabrovšek, F., 2013. Influence of CO2 dynamics on the longitudinal variation of incision rates in soluble bedrock channels: Feedback mechanisms. Geomorphology 186, 85–95.
Fairchild, I., Baker, A., 2012. Speleothem Science: From Process to Past Environments, Quaternary geosciences series.
Gabrovšek, F., Peric, B., Kaufmann, G., 2018. Hydraulics of epiphreatic flow of a karst aquifer. J. Hydrol. 560, 56–74.
Goldscheider, N., Chen, Z., Auler, A.S., Bakalowicz, M., Broda, S., Drew, D., Hartmann, J., Jiang, G., Moosdorf, N., Stevanovic, Z., Veni, G., 2020. Global distribution of carbonate rocks and karst water resources. Hydrogeol. J. 28, 1661–1677.
James, E.W., Banner, J.L., Hardt, B., 2015. A global model for cave ventilation and seasonal bias in speleothem paleoclimate records. Geochemistry, Geophys. Geosystems 16, 1044–1051.
Johnson, M.S., Billett, M.F., Dinsmore, K.J., Wallin, M., Dyson, K.E., Jassal, R.S., 2010. Direct and continuous measurement of dissolved carbon dioxide in freshwater aquatic systems—method and applications. Ecohydrology 3, 68–78.
Kaufmann, G., Gabrovšek, F., Turk, J., 2016. Modelling flow of subterranean Pivka river in Postojnska jama, Slovenia. Acta Carsologica 45, 57–70.
Kaufmann, G., Mayaud, C., Kogovšek, B., Gabrovšek, F., 2020. Understanding the temporal variation of flow direction in a complex Karst system (Planinska Jama, Slovenia). Acta Carsologica 49, 213–228.
Kaufmann, G., Mayaud, C., Kogovšek, B., Gabrovšek, F., 2020. Understanding the temporal variation of flow direction in a complex Karst system (Planinska Jama, Slovenia). Acta Carsologica 49, 213–228.
Kowalczk, A.J., Froelich, P.N., 2010. Cave air ventilation and CO2 outgassing by radon‐222 modeling: How fast do caves breathe? Earth Planet. Sci. Lett. 289, 209–219.
Krajnc, B., Ferlan, M., Ogrinc, N., 2017. Soil CO2 sources above a subterranean cave—Pisani rov (Postojna Cave, Slovenia). J. Soils Sediments 17, 1883–1892.
Kukuljan, L., Gabrovšek, F., Covington, M.D., Johnston, V.E., 2021a. CO2 dynamics and heterogeneity in a cave atmosphere: Role of ventilation patterns and airflow pathways. Theor. Appl. Climatol. 146, 91–109. Javni razpis za (so)financiranje raziskovalnih projektov za leto 2022
Kukuljan, L., Gabrovšek, F., Covington, M.D., 2021b. The relative importance of wind‐driven and chimney effect cave ventilation: Observations in Postojna Cave (Slovenia). Int. J. Speleol. 50, 275–288.
Kukuljan, L., Gabrovšek, F., Johnston, V.E., 2021c. Low‐calcium cave dripwaters in a high CO2 environment: Formation and development of corrosion cups in postojna cave, slovenia. Water 13, 8–16.
Lang, M., Faimon, J., Godissart, J., Ek, C., 2017. Carbon dioxide seasonality in dynamically ventilated caves: the role of advective fluxes. Theor. Appl. Climatol. 129, 1355–1372.
Liu, Z., Macpherson, G.L., Groves, C., Martin, J.B., Yuan, D., Zeng, S., 2018. Large and active CO2 uptake by coupled carbonate weathering. Earth‐Science Rev. 182, 42–49.
Mattey, D.P., Atkinson, T.C., Barker, J.A., Fisher, R., Latin, J.P., Durrell, R., Ainsworth, M., 2016. Carbon dioxide, ground air and carbon cycling in Gibraltar karst. Geochim. Cosmochim. Acta 184, 88–113.
Milanolo, S., Gabrovšek, F., 2015. Estimation of carbon dioxide flux degassing from percolating waters in a karst cave: Case study from Bijambare cave, Bosnia and Herzegovina. Chemie Der Erde‐Geochemistry 75, 465–474.
Noronha, A.L., Johnson, K.R., Southon, J.R., Hu, C., Ruan, J., McCabe‐Glynn, S., 2015. Radiocarbon evidence for decomposition of aged organic matter in the vadose zone as the main source of speleothem carbon. Quat. Sci. Rev. 127, 37–47.
Prelovšek, M., 2012. The Dynamics of the Present‐Day Speleogenetic Processes in the Stream Caves of Slovenia, ZRC SAZU.
Prelovšek, M., Šebela, S., Turk, J., 2018. Carbon dioxide in Postojna Cave (Slovenia): spatial distribution, seasonal dynamics and evaluation of plausible sources and sinks. Environ. Earth Sci. 77.
Sánchez‐Cañete, E.P., Oyonarte, C.,
Serrano‐Ortiz, P., Curiel Yuste, J., Pérez‐Priego, O., Domingo, F., Kowalski, A.S., 2016. Winds induce CO2 exchange with the atmosphere and vadose zone transport in a karstic ecosystem. J. Geophys. Res. Biogeosciences 121, 2049–2063. Serrano‐Ortiz, P., Roland, M., Sanchez‐Moral, S., Janssens, I.A., Domingo, F., Goddéris, Y., Kowalski, A.S., 2010. Hidden, abiotic CO2 flows and gaseous reservoirs in the terrestrial carbon cycle: Review and perspectives. Agric. For. Meteorol. 150, 321–329.
White, W.B., 2013. Carbon fluxes in karst aquifers: sources sinks, and the effect of storm flow. Acta Carsologica 42(2– 3), 177–186.
Wood, W.W., 1985. Origin of caves and other solution openings in the unsaturated (vadose) zone of carbonate rocks: A model for CO2 generation. Geology 13, 822–824.